A planetary dynamo may be a key factor in creating the conditions needed for life. And creating that dynamo seems to depend on the radioactive decay of thorium and uranium, generating internal heating and driving plate tectonics. Let’s carry this line of thought further, though, as the authors of a new paper out of UC-Santa Cruz do, and point out that these heavy elements are necessary to create a magnetic field like Earth’s, which protects us from damaging radiation.
In the rocky planets, magnetic fields are generated by convection in a metallic core, which in turn is driven by heat extracted into the mantle (Nimmo 2015; Labrosse 2015; Boujibar et al. 2020). Since mantle radiogenic heat production controls how much heat is extracted from the core, it will also influence the presence or absence of a dynamo. Similarly, heat production will control the mantle temperature and thus the rate of silicate melting and volcanism.
That quote is from the paper, whose lead author is Francis Nimmo, and it points to the key role of radiogenic heat in the mantle. Within the Earth, there are enough radioactive elements to generate a persistent ‘geodynamo,’ in which molten iron and nickel in motion in the planet’s outer core produce electric currents, forming a magnetic field as the Earth rotates. The authors find that Earth “had just enough radiogenic heating to maintain a persistent dynamo.” And that has implications for the atmosphere.
UC-Santa Cruz’ Natalie Batalha is director of the university’s Astrobiology Initiative, whose multidisciplinary work is designed to produce the kind of widespread collaboration found in this paper. Says Batalha:
“It has long been speculated that internal heating drives plate tectonics, which creates carbon cycling and geological activity like volcanism, which produces an atmosphere. And the ability to retain an atmosphere is related to the magnetic field, which is also driven by internal heating.”
To ponder how all these effects come together, we have only to look at Mars, where the lack of a magnetic field can be tied to the gradual loss of an atmosphere that was once thick enough to support water on the surface and, potentially, a living environment. The planet’s low gravity was one factor in the gradual thinning of the atmosphere, but the solar wind of charged particles from the Sun is likewise a problem, for without a protective magnetic field, the atmosphere erodes.
We have an angle here into habitability on planets around other stars, for the amount of radioactive material is going to vary from one stellar system to another. The so-called r-process elements — created by nuclear reactions that are responsible for producing roughly half of the atomic nuclei heavier than iron — owe their origin to supernova events and the mergers of neutron star remnants, events that will be stochastic and result in planets with varying degrees of radiogenic materials. Back to the paper on this point:
From the point of view of long-term radiogenic heating, the most important elements are potassium, thorium, and uranium. Th and U are both r-process elements, most likely produced in neutron star mergers (NSMs) (Kasen et al. 2017; Cowan et al. 2019), with possibly significant contributions also from massive star collapse explosions (Siegel et al. 2019; Macias & Ramirez-Ruiz 2019). Because such events are rare (an occurrence rate of tens/Myr in our Galaxy) and produce large quantities of r-process elements, the resulting concentration of r-process elements should vary considerably. This is especially true of low-metallicity stars, because turbulent mixing of the interstellar medium is inefficient in the early Galaxy (Shen et al. 2015; Naiman et al. 2018). This expectation is in agreement with measurements of r-process europium in low-metallicity stars (e.g., Macias & Ramirez-Ruiz 2018) and with r-process abundances in dwarf galaxies (e.g., Ji et al. 2016).
Lead author Francis Nimmo realized that if planets vary in the amount of radioactive elements, we should then expect geological activity and magnetic fields to vary as well. The UC-Santa Cruz scientists essentially adjusted radiogenic heat production in their models to see how this factor affected planetary habitability. As Nimmo puts it:
“We took a model of the Earth and dialed the amount of internal radiogenic heat production up and down to see what happens. Just by changing this one variable, you sweep through these different scenarios, from geologically dead to Earth-like to extremely volcanic without a dynamo.”
When radiogenic heating is dialed up higher than the Earth’s, no dynamo is produced. With most of the thorium and uranium in the mantle, the excessive heat becomes an insulator, so that the loss of heat from the molten core is slowed. The result: Heat loss from the core cannot generate convection strong enough to produce a magnetic field, with all that implies about the survival of an atmosphere and radiation flux on the surface.
Given all that, the associated volcanic activity produced by greater levels of radiogenic internal heating is almost lost in the shuffle, but it could obviously be a factor in mass extinction events. And while that implies that high levels of radiogenic heating are a key factor in suppressing a habitable environment, the study also shows that too little heat results in no volcanism whatsoever. We wind up with a planet that, like Mars, appears geologically dead.
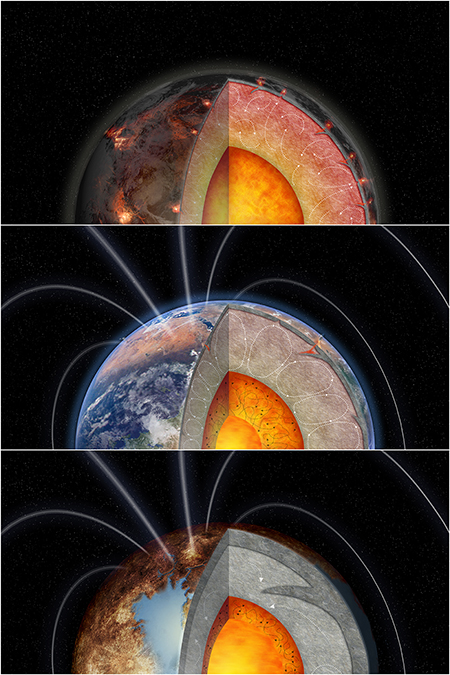
A new study identifies internal heating from radioactive decay as a critical factor in a planet’s ability to generate a magnetic field and retain an atmosphere. Credit: Illustration by Melissa Weiss for UC Santa Cruz
Image: These illustrations show three versions of a rocky planet with different amounts of internal heating from radioactive elements. The middle planet is Earth-like, with plate tectonics and an internal dynamo generating a magnetic field. The top planet, with more radiogenic heating, has extreme volcanism but no dynamo or magnetic field. The bottom planet, with less radiogenic heating, is geologically “dead,” with no volcanism. Caption credit: UC-Santa Cruz.
So we are working with a single variable, one that can be studied via spectroscopy to measure the abundance of the various elements in stars, the assumption being that planets will have the same composition as the stars they orbit. Here the rare earth element europium comes into play, readily observed in stellar spectra, and created by the same processes that produce thorium and uranium. In this work, europium is the tracer for studying radiogenic abundance.
We already have europium measurements for many nearby stars, which allowed Nimmo and team to set a range of inputs for their models of radiogenic heating, with the Sun’s composition establishing the middle of the range. Europium thus becomes a measurement that can flag radiogenic elements in exoplanetary systems as we look forward to the deployment of the James Webb Space Telescope. The europium measurement may well show us which stellar systems are likely to produce planets with the internal composition life would need to survive. From the paper:
…higher mantle heating rates increase volcanic activity and shut off the dynamo, both likely deleterious for habitability. In contrast, reduced mantle heating rates will at some point stop melt production. A potential consequence is that plate tectonics will then cease (Louren¸co et al. 2018), and with it, the dynamo, as at Venus (Nimmo 2002). It is tempting to speculate that the Earth is habitable in part because it possesses a “Goldilocks” concentration of radiogenic elements: high enough to permit long-lived dynamo activity and plate tectonics, but not so much that extreme volcanism and dynamo shutoff occur. As yet, however, our understanding of the complicated feedbacks involved is too rudimentary to make such a definitive conclusion; more detailed calculations, and better characterization of the radiogenic element abundances in planet-hosting stars, are both required.
The paper is Nimmo et al., “Radiogenic Heating and Its Influence on Rocky Planet Dynamos and Habitability,” Astrophysical Journal Letters Vol. 903, No. 2 (10 November 2020). Abstract / Preprint.
Radiogenic Double edged sword.
This feels like one of the strong filters preventing ETIs from arising.
If the Earth-Theia Collision had not happened would the Earth’s
core have enough radiogenic potential to keep the interior active to
our present time.
If the Core is too large, does it create so much crustal instability that
land animals get continually wiped out every 100 million years or so.
I think a very fine tuning is necessary, to get it just right.
IMO the Earth has a bit too much tectonic activity so a core of 5-10%
smaller would be a benign alteration, But that would mean that the core might go dormant in the next few hundred million years.
If that is the case, then we have yet another tuning variable that makes Earth a rarer environment that expected. If the hypothesis is correct, it should be testable against a catalog of biosignatures sometime this century.
Elsewhere the text indicates that the radiogenic elements have higher abundences towards the outer edge of the galactic disc. This would imply life is more common here, compared to the galactic core. So no galactic civilization there unless it has migrated to that region for other reasons.
It will be interesting to follow this line of research as teh models get better to determine how critical this is, and whether there are mitigating factors or not. Astro-engineering civilizations may need to install magnetic fields to ensure that their terraformed worlds retain their precious atmospheres over the long term. Would such machines be detectable from Earth?
Well, I think we all like fairy tales but when too many criteria make the story sound ow so real, there is a fly in the soup. Here is another one, the earth is the only body in the solar system the has an equal number of total solar to annular eclipses. What, you say, does this have to do with anything? Well, this is the how limited we are in understanding what actual exist in the universe. The rare earth mentality is like any other psychoses, sorry to say, but nature has a lot of dirty tricks up its sleeve, like Covid-19. When we fixate on only things close to us we loose our ability gain perspective on the subject. We need to look to the early stars that date back to the beginning of time (if there is a beginning) to see just what actual took place.
HD 140283.
https://en.wikipedia.org/wiki/HD_140283
The older then time, great great grand daddy, of all stars a nice G0 and only 200 LYs away!
HD 175607.
http://www.openexoplanetcatalogue.com/planet/HD%20175607%20b
2MASS J18082002?5104378.
https://en.wikipedia.org/wiki/2MASS_J18082002%E2%88%925104378
My earlier comments in “On 300 Million Habitable Zone Planets.”
Michael Fidler November 10, 2020, 22:17
“These early stars especial M Dwarfs would have no or very little radioactive elements in the sense of nuclear destruction. We live on an overly dense planet and most of the early Planets would be made of biologically important organic elements. This would seem to be the best long term environment for life and the majority of these stars are in Halo orbits. This should lead to much calmer and and less flaring in their central stars. I would be inclined to say that first five billion years of our Galaxy would be a much better place for life and civilizations.”
Michael Fidler November 11, 2020, 10:25
“Here is an opposing opinion that I came across this morning:
Radioactive elements may be crucial to the habitability of rocky planets.
Earth-size planets can have varying amounts of radioactive elements, which generate internal heat that drives a planet’s geological activity and magnetism.
https://news.ucsc.edu/2020/11/planet-dynamos.html
Radiogenic Heating and its Influence on Rocky Planet Dynamos and Habitability.
https://arxiv.org/abs/2011.04791
This makes sense since we live on the densest planet in the solar system, but again we see it from the earth center point of view.
We do have a lower density body in this solar system that has many active volcanoes and that is Jupiter’s Io. The early planets around red dwarfs may of had little thorium, and uranium to cause radiogenic heating but because of there close proximity to the central star and other planets they may have been very active. The high magnetic field generated by red dwarfs plus the tidal heat caused by both the star and planets passing near each other would cause heating and internal magnetic fields. Induction heating may keep plate tectonics active on these early planets and kept them habitable.
Interior Structures and Tidal Heating in the TRAPPIST-1 Planets.
https://arxiv.org/abs/1712.05641
Solid tidal friction in multi-layer planets: Application to Earth, Venus, a
Super Earth and the TRAPPIST-1 planets. Can a multi-layer planet be approximated as a homogeneous planet?
https://arxiv.org/abs/2010.04587
Magma oceans and enhanced volcanism on
TRAPPIST-1 planets due to induction
heating.
https://arxiv.org/abs/1710.08761
So again we look at the even early planets as being lifeless and dead but we have our blinders on and can not see natures beauty.”
The argumentation related to the absence of magnetic field of planets that have too hot core makes whole article very doubtful…
We need more observation of ET planets , including real data about magnetic field of ET planets.
Do not think that it is correct approach to ignore strong magnetic fields of stars and gas giants, limiting theory only by rocky bodies.
Early Earth had a higher concentration of uranium 238 (4 Gy half life), and had larger amounts of residual heat in the core and mantle. It would be interesting to see whether this affected Hadean Earth’s magnetic field.
Presumably, order of magnitude differences under study here, so that may or may not be relevant.
Has it not been accepted that a natural (of course) nuclear reactor is alive and well at the earth’s core? From various articles, this reactor apparently accounts for 50% of nuclear-related heat generation with the balance from radioactive decay.
The reactor’s existence was proved by detection of associated neutrinos:
https://blogs.scientificamerican.com/observations/nuclear-fission-confirmed-as-source-of-more-than-half-of-earths-heat/
The highly variable geomagnetic field can be explained by variable output to the reactor:
https://www.pnas.org/content/98/20/11085
Despite the confirmation of the reactor’s existence, it seems to be ignored by many subsequent papers such as the one referenced in the posting.
Those who have accepted the reactor’s existence have proposed that similar reactors could exist at the core of other planets and even as the fission trigger of thermonuclear fusion in low mass stars.
Perhaps poor old Mars did not have conditions suitable for the formation of a nuclear reactor or its reactor shutdown early in its history.
This concept is so cool and confirmed yet seems often ignored in some research papers.
As you suggest in your comment. The top panel of the
e paper showing temperatures seems to contradict the PNAS figure 1 on temperatures. Are the models different due to the PNAS hypothesis of fission, whilst the Nimmo paper assumes only radiogenic decay? Has the PNAS paper hypothesis been confirmed by others, or is it flawed and been abandoned?
The neutrino evidence for nuclear fission at the earth’s core would seem solid to whatever the error margin is for such measurements. The core issues (ha ha) is the presence of a significant (and variable) heat source at the center of the earth. It would seem that the neutrino evidence is hard to reconcile with any other explanation. For what my opinion is worth in these matters, the authors of the present paper need to broaden their analysis to include these “geo-reactors”. One could speculate that lots of elements for radioactive decay would indicate a lot of fissionable materials as well. Assuming that these elements can concentrate at the planetary core, a very strong source of fission-generated heat will be present resulting in a planet with a great deal of volcanism and a strong magnetic field.
Could be a reason that Venus erupted huge amounts of lava, there may have been more nuclear material in it core causing it to insulate and in a huge event attempt to turn over the heat building up in the mantle.
Mars has lost it’s heat because it is smaller and has less radioactive elements, a much smaller planet will cool off faster due to it’s smaller size. We should look at all G class stars in case the rare element idea does not apply.
I would think a hotter core would give a stronger magnetic field with more fluidity which could be dependent on the size of the planet after the giant impact which give a planet it’s angular momentum so I agree with this paper.
Exoplanets in the goldilocks zone around M dwarfs and smaller K stars are tidally locked as written in a previous article here on Centauri Dreams. Consequently, due to the exoplanet around these stars being inside the Hill sphere, they can’t have a Moon like our Earth, so the Earth as the center of the solar system or Geocentric model does not apply to M dwarfs.
The idea that the our Earth has a large, hot core is based on the giant impact hypothesis, so the idea of having the right amount of radioactive elements is contingent on that theory in other words a small difference in density counts for a lot or indicates the collision. Density of Venus 5.24 g/cm³ and the density of Earth. 5.51 g/cm³ , A rare Earth would need a magnetic field, and a lot of angular momentum.
Consequently, the idea that all exoplanets with indigenous ET humanoid like must have a Moon is not limited to the Geocentric or prescientific world view, i.e., there are scientific reasons for the need of a Moon and solar system similar to our own in order life to evolve with it’s full genetic potential like here on Earth.
The giant impact may be the reason for more geologically active systems since all the large planets have had them. Even Venus may of been impacted but to the detriment of its rotation. The M dwarfs will not need moons because the small distances between each planet will have the same effect as our moon. This is why the need for a large moon is purely because we have the one and only one such system. The scientific method works because the only way the proof works is by enough samples and ours is limited to one. The long term results will show many cases that have similar results created by many different combinations and since M dwarfs dominate the star systems that is where the most combinations will be. We have limited data, we do not have a rare earth, but an ancient earth centered mentality. The genetic potential and solar systems similar to our own is a fallacy with no scientific backing. What little real data is being used for public and financial backing as we deal with a world wide population that has limited knowledge. Earth 2.0 is a prime example of fake news since no planets will be the same as earth. I think all the advance civilization’s out there realized that it will be only a matter of time before we will realize this is the situation. The question is when will the scientific community start to work for a world wide system of public education on what is out there instead of feeding the them the religious point of view.
Not to beat the geo-reactor theory into the ground, but it offers another variable in estimating the habitability of planets insofar as the impact of a long-lasting planetary magnetic field. If high metallicity suggests a high concentration of fissionable elements are present during planetary formation, then the potential for fission reaction in planetary cores may be high. Smaller planets (Mars size) around G class starts or planets in the habitable zones around red dwarfs may have sufficiently strong magnetic fields for protection of the planetary atmosphere.
Does Venus have a geo-reactor?
In any event, the area of nuclear fission in celestial bodies needs more attention I believe.
Let’s see…
We need an old, stable main sequence star, preferably one born in a time and place where the interstellar medium had been enriched with metals but not too close to high radiation environments such as black holes or the galactic center. And we need a sun without an erratic orbit around the galactic center and far enough away from any other cosmic catastrophes or near misses..
We also need a star with a big habitable zone, but no flares or other coronal activity that might injure life. And it will help if the planet is not tidally locked to its primary and has a thick atmosphere well stocked with ozone in its upper layers .
In addition, we need a planetary system that is not perturbed by marauding gas giants, but still has enough of them to sweep clean any potential colliders.
Oh, and lets not forget we need at least one collider to get through to give us a moon, because tides are essential to mix up the oceans and keep planetary rotation stable and the obliquity of the ecliptic within narrow bounds!
We need plate tectonics, a magnetic field, a metallic, radioactive core to drive the whole thing, and THEN….nearly five billion years of stable conditions to nurture life.
And if, and only if, we have ALL of these unlikely preconditions, then we will get life, metazoa, intelligence and a technological culture. Guaranteed. We must be the only planet in the galaxy to meet all these conditions, so we must be the only one to have sapient creatures and a civilization on it. Isn’t it wonderful that the only world capable of having a space faring civilization is ours, and we were born into it just in time to participate?
For those of you who are impervious to sarcasm, I suggest you go through all the Goldilocks delusions again and see if they still make sense to you.
Life is tough, its adaptable, and its resilient. I suspect it will show up and flourish whenever and wherever the conditions are not impossible. If we keep on hoping for reasons why it can’t arise we may just convince ourselves that we are impossible and only a supernatural intervention allowed us to arise, which is what all these anthropic speculations seem to be demanding of us. I don’t know about you, but I don’t want to live in a universe that depends on supernatural interventions. I prefer the Principle of Mediocrity.
“That which is not forbidden is mandatory.”
Do not forget the golf course!
Agree with your conclusion (and that of Michael Fidler) that we are probably not so special or unique in having (higher) life.
What is disagree with is your statement ‘ALL of these unlikely preconditions’.
Most of those conditions are coinciding: a G class star usually has certain characteristics, and so, probably, does a terrestrial planet: iron core, radioactive mantle, plate tectonics and magnetic field largely go together.
The large impactor/large moon may be the exceptional thing, but as I have pointed out several times, the importance of that for stabilizing planetary tilt has often been overrated (see Barnes et al.).
What this post and referred paper are about, is the proper amount of radioactive elements in the mantle, which is most likely a range, not an exact value.
We see more and more that life depends on, and can exist within, ranges of parameter values, like a zone, with possibly an optimum (super-habitability) and extremes, where only extremophiles can exist.
What a way to spice up the Universe: “turbulent mixing of the interstellar medium is inefficient in the early Galaxy”! With this little sentence, we may have the frequency of intelligent life recede by orders of magnitude, but we populate the galaxy with a range of environments and (perhaps) biochemistries more suited to our fervid imagination.
The preprint with its 1D model sounds only a step up from the back of the envelope, but it is only a plea for data after all. The interaction of r-process elements with thermal conductivity, the presence of plate tectonics, and I assume planetary radius, equilibrium temperature and mantle viscosity… I doubt it all works out to a simple Galactic Habitable Zone. There is a tendency to be jaded when there are more exoplanets in a survey than raisins in a box, yet each of these is an unknown world with all the mystery and majesty that entails.
During each transit, starlight streams through an exoplanet’s magnetic field and Van Allen belts. Is there an instrument powerful enough to see the effects of this? I see news stories that say it can’t be done, also this one that did it ( https://dailygalaxy.com/2019/07/exoplanet-magnetic-fields-detected-200-times-earths/ ) but only for a gas giant with a field of 120 gauss. It’s a start…
This takes a big bite out of that 300 million planets. The narrow range of water densities hospitable to life takes another big bite. The collection of elements required for life takes one too. Can’t take many more bites before Life is rare and complex life is common. Forgive my hubris but I think humanity’s existence predicts many planets with complex life.
Life started quickly on Earth. I would expect the same for any planet with a magnetic field, plate tectonics, optimal water densities, and the elements required for life. Life looks so powerful when viewed as a symmetry of thermal dynamic engineering and genetic information processing.
We shouldn’t have to reproduce the Moon forming event to get a suitable planet. There would be a range of scenarios that could deliver the sufficient conditions. If a planet spins fast enough it can keep a stable tilt without a moon. I could imagine complex life on wobbly planets as well. Could there be system configurations that don’t produce a lot of jostling?
“If a planet spins fast enough it can keep a stable tilt without a moon.”
Exactly! The importance of a large moon for axial tilt stabilization is often grossly overrated, the gyroscopic effect of the spinning planet itself is at least as, or more important.
See for instance Lissauer, Barnes et al. 2011: Obliquity variations of a moonless Earth.
Just to say, a planet with too much radioactivity will eventually be a planet with just the right amount of radioactivity.
I was thinking the same. Might be especially relevant to planets orbiting smaller stars with long stellar lifetimes.
I’ll also add that more volcanism might balance out the atmospheric erosion on planets without magnetic fields. And that Venusian habitability problems are not caused by having too little of an atmosphere.
Finally, mass extinctions may be problematic for complex life but maybe not so much for microbes. It would take quite the volcano to sterilize a planet completely.
Talking about a hot subject:
This asteroid just skimmed Earth’s atmosphere.
Just 238 miles or 383 km over Earth’s surface, on Friday the 13th no less!!!
https://earthsky.org/space/asteroid-2020-vt4-skimmed-atmosphere-fri-nov-13-2020
I had just watched the movie Greenland about a giant interstellar comet destroying the earth and woke up to this the next morning!!!
Take a look at the orbit simulator of the path!!!
http://orbitsimulator.com/gravitySimulatorCloud/simulations/1605380216698_A10sHcN.html
Well, it past thru the area that most of the SpaceX Starlink satellites are in. In the not too distant future there could be well over 50,000 internet satellites at that height and one direct hit would cause a berserker cascade of impacts. Maybe SpaceX and the other companies that plan on internet constellations should fund a better detection systems (telescopes) so that they do not learn of the impacts until the next morning…
Yes, a lot of contingences for life to evolve on an exoplanet “takes big bite out of 300 million. There is also the limitation to G class stars and large K stars which don’t have tidal locking, so exoplanets can hold onto an atmosphere over time since they have fast rotation and magnetic field. I don’t think these contingences support the rare Earth hypothesis with only one planet in the whole galaxy with life, but only a semi rare Earth hypothesis.
Even with a lot of contingences, an ultra conservative view might predict that in potential there could be from ten to fifty exact Earth twins with a Moon, etc. on our side of the galaxy which host indigenous ET life. This is based on probability, or how G class systems protoplanetary disks form which ends up with a lot of potentials to make Earth twins and have a giant impactor in the same orbit or very close orbit. We could still conclude that the anthropomorphic principle is valid and our universe is fine tuned for humanoid life.
“There is also the limitation to G class stars and large K stars”.
No, the estimated 300 million *is* the number of terrestrial planets orbiting in the HZ of solar type stars.
I.e. the steller type limitation is already in there.
Hi Paul
I was looking forward to reading your post on this topic, It sure is very interesting and another variable to consider on Habitability of a terrestrial planet.
I now need to read the paper and also the one mention in your other recent post too.
Cheers Laintal
A Sunlight Shifted Asteroid Might Hit the Earth in 2068 with a 1200 Megaton Impact
Dave Tholen and collaborators have detected light from the sun is moving the 300 meter near-Earth asteroid Apophis by 170 meters per year. This acceleration means that Apophis might hit the earth in 2068.
Detailed analysis on the next close pass in 2029 will determine if Apophis is a risk.
The Sentry Risk Table estimates that Apophis would impact Earth with kinetic energy equivalent to 1,200 megatons of TNT.
The highest probability of impact is on April 12, 2068 and the odds of an impact on that date, as calculated by the JPL Sentry risk table using a March 2016 solution, are 1 in 150,000. The calculations are that it should miss the Earth by 150 million kilometers. The acceleration should only shift the asteroid by about 122 kilometers.
Assuming Apophis is a 370-meter-wide (1,210 ft) stony asteroid with a density of 3,000 kg/m3, then if it were to impact into sedimentary rock, Apophis would create a 5.1-kilometer (17,000 ft) impact crater.
A deep-water impact in the Atlantic or Pacific oceans would produce an incoherent short-range tsunami with a potential destructive radius (inundation height of about 2 meters) of roughly 1,000 kilometers (620 mi) for most of North America, Brazil and Africa, 3,000 km (1,900 mi) for Japan and 4,500 km (2,800 mi) for some areas in Hawaii.
As others have noted, Venus is the obvious counterpoint to the atmosphere erosion & magnetic fields theories. I suspect that this might be a size issue. Atmospheric erosion is significant for planets masses smaller than a critical size thats intermediate between Earth & Mars.
One other aspect of this theory, if it does hold for Earth size planets, is that for Super Earths, a degree of atmospheric erosion might make their atmospheres more suitable for life (as we know it).
The problem with the super Earth’s is they have too much gravity and probably don’t have Moons, an intuitive hypothesis. We’ll look there for life anyway though.
There might indeed be some super Earth’s with a very Earthlike atmosphere and surface temperature and even with Moons, but whether or not intelligent life like us has evolved there remains to be seen.
“We could still conclude that the anthropomorphic principle is valid and our universe is fine tuned for humanoid life.” Are we that conceited? This is the reason, a closed mind set is the worst possible way to see the universe and nature. This Disney point of view is childish and if we ever expect to make any type of contact a much border viewpoint needs to be made. Since humans tend to believe we are the crown of creation maybe A.I. needs to be used to see a more balanced view of intelligent life’s possibilities. Just let them read a few of the more insightful science fictions…